INTRODUCTION
The human body is a complex system of natural enzymatic and non-enzymatic antioxidant defenses which counteract the harmful effects of free radicals and other oxidants. Free radicals are reported for causing various diseases including cancer, cardiovascular disease, neural disorders , Alzheimer’s disease, mild cognitive impairment, Parkinson’s disease , alcohol induced liver disease, ulcerative colitis, aging and atherosclerosis . Protection against free radicals can be enhanced by antioxidants. There are several evidences which prove that foods containing various antioxidants are of major importance in disease prevention. The present review explains the concept of free radicals and antioxidants.
1. FREE RADICALS
By definition a free radical is any atom (e.g. oxygen, nitrogen) with at least one unpaired electron in the outermost shell and is capable of independent existence. A free radical is easily formed when a covalent bond between two entities is broken and one electron remains with each newly formed atom. Free radicals are highly reactive due to the presence of unpaired electron(s). Generally two types of free radicals; reactive oxygen species (ROS) and reactive nitrogen species (RNS) are involved in human physiology. Any free radical involving oxygen can be referred to as reactive oxygen species (ROS) and free radical involving nitrogen is referred as reactive nitrogen species (RNS). Free radicals present a paradox in their biological function: on one hand, they protect from diseases by assisting the immune system, mediating cell signaling and playing an essential role in apoptosis. On the other hand, they can damage important macromolecules in cells and may have a role in aging, carcinogenesis and cardiovascular diseases [1,2].
1.1 Reactive oxygen species (ROS)
The chemical biology of dioxygen encompasses a large variety of reactions; most of them are highly beneficial to the organisms but some of them are deleterious. Dioxygen, is a powerful oxidizing agent and is the energy that fuels most non-photosynthetic biology. It must be supplied continuously to respiring cells. Dioxygen is also used as a source of oxygen atoms in a large variety of enzyme-catalyzed biosynthetic reactions of organic substrate molecules. The same oxidizing capacity of oxygen is the basis of respiration, however, also makes dioxygen simultaneously an agent of toxic oxidative stress [3].
The dioxygen molecule is in two unpaired electrons in its most stable form. Consequently, their direct reactions with other molecules are generally slow in the absence of catalysts or radical initiators and are therefore not the primary causes of oxidative stress. The superoxide, hydrogen peroxide, organic peroxides, hydroxyl radical, peroxynitrite, and other energetic molecules derived from them further appear to be the agents of oxidative damage [4]. These molecules or ions are collectively termed 'reactive oxygen species', or 'ROS'. Their ultimate source is found to be in mitochondria, where side reactions of dioxygen with components of the respiratory chain reduce it to superoxide. Superoxide may itself cause damage or may react further to give other ROS [5].
Types of reactive oxygen species (ROS)
Research also demonstrates that ROS generation is a normal physiological process, particularly for proper immunocompetence and in coordination and activation of numerous signal transduction pathways [6]. The formation of ROS is a natural consequence of aerobic metabolism and is integral for maintaining tissue oxygen homeostasis [7]. Oxygen homeostasis (the balance between constitutive oxidants and antioxidants) is maintained through a natural series of reduction– oxidation (redox) reactions which involve in the transfer of electrons between two chemical species: compounds that lose electrons (oxidized) and those that gain electrons (reduced). When oxygen homeostasis is not maintained, the cellular environment becomes oxidatively stressed. Approximately 1–3% of oxygen is used by the body get converted into ROS [8]. Three of the major ROS (superoxide radical, hydrogen peroxide and hydroxyl radical) are normally produced during metabolism by the mitochondria in growing cells [4]. Other significant intracellular sources of ROS include microsomal cytochrome P450 enzymes, flavoprotein oxidases and peroxisomal enzymes involved in metabolism of fatty acid [9]. Potentially damaging oxidative stress can be generated by excess ROS, which are in control by endogenous cellular antioxidant mechanisms. Oxidative stress-related enzymes include superoxide dismutases for eliminating the superoxide radical as well as catalase and glutathione peroxidases for removing hydrogen peroxide and organic peroxides [7]. Transient fluctuations of ROS levels influence activity of signal transduction pathways leading to cell proliferation, apoptosis or necrosis, depending on the dosage and duration of ROS and also on cell type. Typically, low doses of ROS can be mitogenic, whereas medium doses lead to temporary or permanent growth arrest (replicative senescence), and high doses usually result in cell death either by apoptosis or necrosis. Although necrosis and apoptosis may be viewed as negative events in terms of cell loss, these processes also have positive roles in the down-regulation of immune responses and elimination of transformed cells (tumor suppression) (Fig. 1) [2].
Superoxide anion: Superoxide anion (O2.-) is an electron reduction product of molecular oxygen. Superoxide anion is also the key player in hydroxyl radical generation in vivo because its dismutation is the primary source of cellular H2O2. It can play the role of reducing agent for catalytic metal ions in the Fenton reaction. Superoxide on a chemical level is a sluggish reactant [5].
Hydroxyl radical (.OH): The hydroxyl radical (.OH), is the neutral form of the hydroxide ion. The hydroxyl radical has a high reactivity with a very short in vivo half life of approx 10-9 s. Thus, when produced in vivo, the .OH reacts close to its site of formation. The redox state of the cell is largely linked to an iron (and copper) redox couple and is maintained within strict physiological limits. It has been reported that iron regulation confirms that there is no free intracellular iron. However, under stress conditions, an excess of super oxide releases “free iron” from iron-containing molecules. The released Fe2+ can participate in the Fenton reaction, generating highly reactive hydroxyl radical (Fe2+ + H2O2 → Fe3++ .OH + OH-). Thus under stress condition, super oxide anion radical facilitates .OH production from hydrogen peroxide by making Fe2+ available for Fenton reaction (O2 .- + H2O2 → O2 + .OH + OH- ) [11].
1.2 Reactive nitrogen species (RNS)
Nitric oxide (NO.) is generated in biological tissues by specific nitric oxide synthases (NOSs). NO . is an abundant reactive radical that acts as an important oxidative biological signaling molecule in physiological processes, including neurotransmission, blood pressure regulation, defense mechanisms, smooth muscle relaxation and immune regulation. Overproduction of reactive nitrogen species is called nitrosative stress. Nitrosative stress may lead to nitrosylation reactions that can alter the structure of proteins and so inhibit their normal function [10].
Cells of the immune system produce both the super oxide anion and nitric oxide during inflammatory processes. Under these conditions, super oxide anion and nitric oxide may react together to produce significant amount of a oxidatively much more active molecule, peroxynitrite anion (ONOO-), which is a potent oxidizing agent that can cause DNA fragmentation and lipid peroxidation (NO.+O2 .-→ ONOO-) [11].
2. Oxidative damage to biological molecules caused by free radicals
At high concentration, ROS can be important mediators of damage to cell structure, nucleic acids, lipids, and proteins (Fig. 1). The hydroxyl radical is known to react with all components of the DNA, damaging both the purine and pyrimidene bases and the deoxyribose backbone resulting in oxidative damage which represents the first step in mutagenesis, carcinogenesis and aging [12]. It is known that metal-induced generation of ROS results in an attack not only on DNA, but also cellular components like poly unsaturated fatty acid residues of phospholipids, which are extremely sensitive to oxidation [13]. How the free radicals cause oxidative damage to biological molecules are describe below:
2.1 Lipids and carbohydrates
Lipid peroxidation not only threatens the integrity and function of membranes and membranous proteins but also produces a variety of toxic aldehydes and ketones, one of the worst i.e. trans-4-hydroxy-2-nonenal (HNE), is produced in high yield [14]. HNE and malondialdehyde (MDA), toxic products formed by peroxidation of lipids, are known to react with side chains of proteins and may result in protein cross-linking. Oxidative damage of carbohydrates produce such products that can be reactive with proteins and result in their damage [15].
2.2 Proteins
Chemical studies of radical-mediated protein oxidation have demonstrated oxidative modification of protein side chains, backbone cleavage, and protein-protein dimerization [17]. Sulfur-containing side chains are particularly vulnerable to oxidation at sulfur, but other oxidative pathways can results to carbonyl-containing products such as aldehydes and ketones which further initiate the peroxidation of lipids [12].
2.3 Nucleic acids
Elevated levels of oxidative stress result in oxidation of DNA and recent results suggest that free intracellular iron is involved in this oxidation [18]. It is a widely known that 'free' iron bind loosely to various sites in the DNA, where it can act as a catalyst for the generation of many reactive species from hydrogen peroxide that reacts with DNA in the immediate vicinity. Hydroxyl radical, an iron ferryl radical and an iron-bound hydroxyl radical are among the species that are suggested to attack DNA [19].
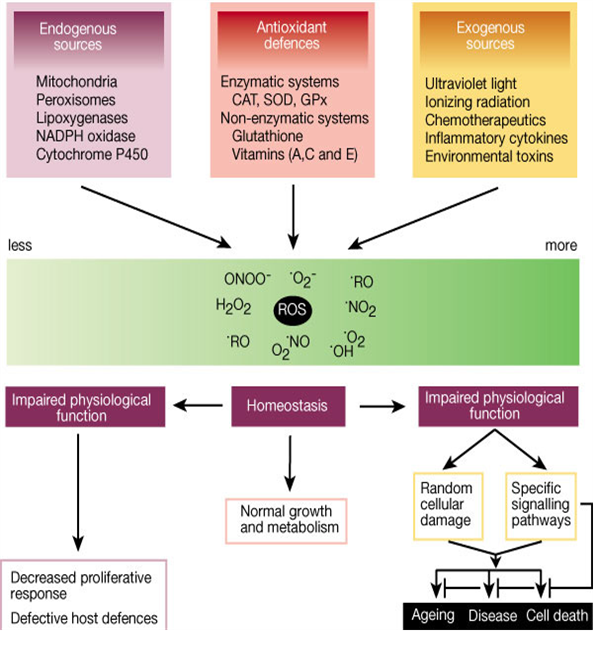
Fig. 1 Role of reactive oxygen species (ROS) [16]
3. Free radical’s involvement in diseases
There are several evidences for the involvement of oxidative damage in several types of diseases, although the identity of the ROS formed, the nature of the chemical reactions involved in their formation and the primary targets of oxidative damage are often not yet identified. In the case of diseases that lead to neurodegeneration, redox metal ion involvement has frequently been implicated sites [20].
The oxidation induced by ROS may result in cell membrane disintegration, membrane protein damage and DNA mutations which play an important role in aging and can further initiate in the development of various diseases, like arteriosclerosis, cancer, diabetes mellitus, liver injury, inflammation, skin damages, coronary heart diseases and arthritis (Fig. 2) [21].
3.1 Aging
Aging is an inevitable biological process that affects most living organisms. The process of aging may be defined as a progressive decline in the physiological functions of an organism after the reproductive phase of life. The free radical theory of aging was first introduced in 1956 by Denham Harman who proposed the concept of free radicals playing a role in the aging process [22]; his work has gradually triggered intense research into the field of role of free radicals in biological systems. Generally, there are two main theories describing the process of aging: damage-accumulation theories and genetic theories. Damage accumulation theories involve “free radical theory”, “glycation theory”, “error catastrophe theory”, “membrane theory”, “entropy theory” and others, among which “free radical theory” is probably the most complex approach to explain the process of aging [23].
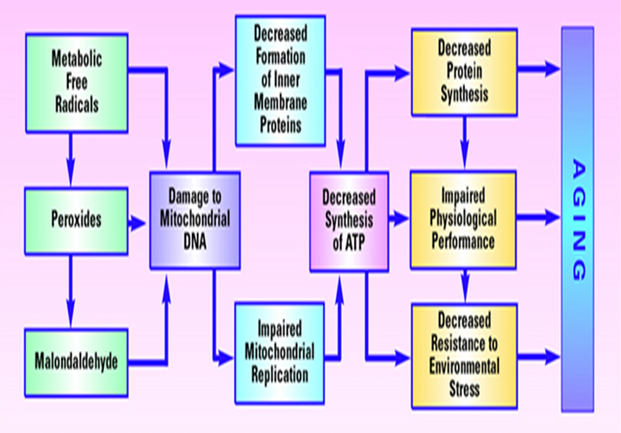
Fig. 2 Model of aging based on free radical-induced damage to mitochondrial DNA[24]
The “free radical approach” is based on the fact of random negative effects of free radicals produced during aerobic metabolism cause damage to DNA, lipids, and proteins and accumulate over time. The aging starts with oxygen, occupying the final position in the electron transport chain [23]. Even under ideal conditions, some electrons “leak” from the electron transport chain. These leaking electrons react with oxygen to produce ROS, under physiological conditions, about 1"3% of the oxygen molecules in the mitochondria are converted into ROS. The main site of damage from ROS is mitochondrial DNA (mtDNA). The cell repairs much of the damage done to nuclear DNA (nDNA), but mtDNA cannot be readily fixed. Therefore, extensive mtDNA damage accumulates over time and shuts down mitochondria, causing cells to die and the organism to age (Fig. 2) [25].
3.2 Cancer
Oxidative stress initiates a cellular redox imbalance which has been found in various cancer cells compared with normal cells; the redox imbalance is may be related to oncogenic stimulation. Permanent modification of genetic material due to “oxidative damage” represents the first step of mutagenesis, carcinogenesis, and aging. DNA mutation is a critical step of carcinogenesis and elevated levels of oxidative DNA damages have been repoted in various tumour cells, strongly implicating such damage in the etiology of cancer events [10,23]. To date, more than 100 oxidised DNA products have been identified. ROS-induced DNA damage involves single- or double-stranded DNA breaks, purine, pyrimidine, or deoxyribose modifications, and DNA cross-links. DNA damage may end in, arrest or induction of transcription, induction of signal transduction pathways, replication errors, and genomic instability, all of which are associated with carcinogenesis [10,15].
3.3 Cardiovascular diseases
The oxidative stress due to ROS in cardiac and vascular myocytes has been linked with cardiovascular tissue injury [26]. Regardless of the direct evidence for a link between oxidative stress and cardiovascular disease, oxidative stress due to ROS plays a role in various cardiovascular diseases such as atherosclerosis, ischemic heart disease, hypertension, cardiomyopathies, cardiac hypertrophy and congestive heart failure. The major sources of oxidative stress in cardiovascular system involve: (i) the enzymes xanthine oxidoreductase (XOR), (ii) NAD(P)H oxidase (multisubunit membrane complexes) and (iii) NOS as well as (iv) the mitochondrial cytochromes and (v) hemoglobin. NOSs and hemoglobin are also principal sources of RNS, including NO• and SNOs (NO-modified cysteine thiols in amino acids, peptides, and proteins), which convey NO• bioactivity [15].
Oxidative stress is associated with increased formation of ROS that modifies phospholipids and proteins leading to peroxidation and oxidation of thiol groups [27]. The assaults by ROS lead to changes in membrane permeability, membrane lipid bilayer disrupn tion and functional modification of cellular proteins. In addition to cellular protein and lipid damage, abnormalities in myocyte function due to increased oxidative stress are considered to be associated with the effects of ROS on subcellular organelles.
4. Need of antioxidants
Since in the late 19th and early 20th century, chemists have studied antioxidants as a loosely defined group of compounds characterized by their ability to be oxidized in place of other compounds present. Their uses range from food storage to the vulcanization of rubber, but it was only later that biologists realized the importance of antioxidants in health with the 1960s publications of vitamins and flavonoids, followed by later research in the 1970s on ascorbic acid (vitamin C), cancer, and the common cold [28].
An antioxidant is a molecule capable of slowing or preventing the oxidation of other molecules. Oxidation is a chemical reaction that transfers electrons from a substance to an oxidizing agent. Oxidation reaction can produce free radicals, which start chain reactions that damage cells. Antioxidants stops these chain reactions by removing or stabilizing free radicals, and inhibit other oxidation reactions by being oxidized themselves [29].
Antioxidant systems exist in cells to protect against ROS. Antioxidants in aqueous compartments, for example the cytosol and the extracellular fluids, consist of low molecular weight antioxidants such as glutathione, ascorbate (vitamin C) and antioxidant enzymes such as superoxide dismutases (SOD), catalases and peroxidases [30].
5. Types of antioxidants
For protecting against oxidative damage, aerobic cells are equipped with a variety of non enzymatic antioxidants and antioxidative enzymes with different functions, by which cellular redox-status homeostasis is ensured through the scavenging of various preformed free radicals [31]. Non-enzymatic antioxidants are represented by ascorbic acid (vitamin C), α-tocopherol (vitamin E), glutathione (GSH), vitamin A, carotenoids, flavonoids, and other antioxidants. The cellular radical-scavenging systems include the enzymes such as superoxide dismutase (SOD), which scavenge the superoxide ion by speeding up its dismutation, catalase (CAT), a haeme enzyme, which removes hydrogen peroxide and glutathione peroxidase (GPX), a selenium-containing enzyme, which scavenges other peroxides as well as hydrogen peroxide. These enzymes form a first line of defense from oxidative stress. If the free radical production becomes more than the capacity of enzymatic system to cope up, then second line of defense (vitamins) may come to rescue. Vitamin A and C quench free radicals by oxidizing and inactivating them [32].
Conclusions
The scientific community reviewed the production of ROS in cells as harmful because aberrant production or incorrect regulation may lead to the development of potentially fatal disorders. With the link between ROS and these diseases established, scientists put much effort into antioxidant research.
References:
1. Hõrak, P., Zilmer, M., Saks, L., Ots, I., Karu, U. and Zilmer, K. (2006). Antioxidant protection, carotenoids and the costs of immune challenge in greenfinches. J Exp Biol 209, 4329-4338.
2. Seifried, H.E., Anderson, D.E., Fisher, E.I. and Milner, J.A. (2007). A review of the interaction among dietary antioxidants and reactive oxygen species. J Nut Biochem 18, 567-579.
3. Valentine, J.S.,Wertz, D.L.,Lyons, T.J.,Liou, L.L.,Goto, J.J. andGralla, E.B. (1998). The dark side of dioxygen biochemistry. Curr Opin Chem Biol 2, 253-262.
4. Pomaki, M., Mota, J., Fuente, M.D.L. and Berger, J. (2005). Effects of thiolic antioxidants on in vitro mouse peritoneal macrophage functions. Com Clin Path 13, 176-181.
5. Dhale, M.A., Divakar, S., Umesh, K.S. and Vijayalakshmi, G. (2007). Isolation and characterization of dihydromonacolin-MV from Monascus purpureus for antioxidant properties. Appl Microbiol Biotechnol 73, 1197-1202.
6. Peng, T.I. and Jou, M.J. (2010). Oxidative stress caused by mitochondrial calcium overload. Annal New York Acad Sci 1201, 183-188.
7. Holbrook, N.J. and Ikeyama, S. (2002). Age-related decline in cellular response to oxidative stress, links to growth factor signaling pathways with common defects. Biochem Pharmacol 64, 999-1005.
8. Drew, B., Phaneuf, S., Dirks, A., Selman, C., Gredilla, R., Lezza, A., Barja, G. and Leeuwenburgh, C. (2003). Effects of aging and caloric restriction on mitochondrial energy production in gastrocnemius muscle and heart. American Jf Physiol Regul, Integ Comp Physiol 284, 474-480.
9. Ihara, N., Kurisawa, M., Chung, J.E, Uyama, H. and Kobayashi, S. (2005). Enzymatic synthesis of a catechin conjugate of polyhedral oligomeric silsesquioxane and evaluation of its antioxidant activity. Appl Microbiol Biotechnol 66, 430-433.
10. Valko, M., Leibfritz, D., Moncal, J., Cronin, M.T.D., Mazur, M. and Telser, J. (2007). Free radicals and antioxidants in normal physiological functions and human disease. The Int J Biochem Cell Biol 39, 44-84.
11. Chatterjee, M., Saluja, R., Tewari, S., Barthwal, M.K., Goel, S.K. and Dikshit, M. (2009). Augmented nitric oxide generation in neutrophils, oxidative and pro-inflammatory implications in hypertension. Free Rad Res 43, 1195-1204.
12. Valentin, H.E. and Qi, Q. (2005). Biotechnological production and application of vitamin E, current state and prospects. Appl Microbiol Biotechnol 68, 436-444.
13. Tiwari, O.P. and Tripathi, Y. (2007). Antioxidant properties of different fractions of Vitex negundo Linn. Food Chem 100, 1170-1176.
14. Nam, S.H., Choi, S. P., Kang, M.Y., Kozukue N. and Friedman, M. (2005). Antioxidative, antimutagenic, and anticarcinogenic activities of rice bran extracts in chemical and cell assays. J Agri Food Chem 53, 816-822
15. Marnett, L.J. (1999). Lipid peroxidation-DNA damage by malondialdehyde. Mut res 424, 83-95.
16. Finkel, T.and Holbrook, N. J. (2000). Oxidants, oxidative stress and the biology of ageing. Nature 408, 239-247.
17. Butterfield, D.A. and Lauderback, C.M. (2002). Lipid peroxidation and protein oxidation in Alzheimer's disease brain, potential causes and consequences involving amyloid beta-peptide-associated free radical oxidative stress. Free Rad Biol Med 32,1050-1060.
18. Ames, B.N., Shigenaga, M.K. and Hagen, T.M. (1993). Oxidants, antioxidants, and the degenerative diseases of aging. Proceeding Nat Acad Sci 90, 7915-7922.
19. Pereira, J.A., Oliveira, I., Sousa, A., Valentao, P., Andrade, P.B., Ferreira, I.C.F.R., Ferreres, F., Bento, A., Seabra, R. and Estevinho, L. (2007). Walnut (Juglans regia L.) leaves, phenolic compounds, antibacterial activity and antioxidant potential of different cultivars. Food Chem Toxicol 45, 2287-2295.
20. Choi, J.H., Ryu, Y.W. and Seo, J.H. (2005). Biotechnological production and applications of coenzyme Q10. ApplMicrobiol Biotechnol 68, 9-15.
21. Scartezzini, P. and Speroni, E. (2000). Review on some plants of Indian traditional medicine with antioxidant activity. J Ethnopharmacol 71, 23-43.
22. Harman, D. (1956). Aging—A theory based on free-radical and radiation-chemistry. J Gerontol 11, 298-300.
23. Valko, M., Izakovic, M., Mazur, M., Rhodes, C.J. and Telser, J. (2004). Role of oxygen radicals in DNA damage and cancer incidence. Mol Cell Biochem 266, 37-56.
24. Fleming, J.E., Miquel, J. and Bensch, K.G. (1985). Age-dependent changes in mitochondria. Molecular Biology of Aging, Plenum, New York, 143-155.
25. Cadenas, E. and Davies, K.J.A. (2000). Mitochondrial free radical generation, oxidative stress, and aging. Free Rad Biol Med 29, 222-230.
26. Dhalla, N.S., Temsah, R.M. and Netticadan, T. (2000). Role of oxidative stress in cardiovascular diseases. J Hypertension 18, 655-673.
27. Molavi, B. and Mehta, J.L. (2004). Oxidative stress in cardiovascular disease, Molecular basis of its deleterious effects, its detection, and therapeutic considerations. Curr Opin Cardiol 19, 488-493.
28. Knight, J. (1998). Free radicals, their history and current status in aging and disease. Annal Clin Lab Sci 28, 331-346.
29. Valentao, P., Fernandes, E., Carvalho, F., Andrade, P.B., Seabra, R.M. and Bastos, M.L. (2002). Antioxidant activity of Hypericum androsaemum Infusion, scavenging activity against super oxide radical, hydroxyl radical and hypo-chlorous acid. Biol Pharma Bull 25, 1320-1323.
30. Fridovich, I. (1997). Superoxide anion radical (O2–), superoxide dismutases, and related matters. J Biol Chem 272, 18515- 18517.
31. Fui, H.Y., Shieh, D.E. and Ho, C.T. (2002). Antioxidant and free radical scavenging activities of edible mushrooms. J Food Lipid 9, 35-46.
32. Bhattacharya, A., Ghosal, S. and Bhattacharya, S.K. (2001). Anti-oxidant effect of Withania somnifera glycowithanolides in chronic footshock stress-induced perturbations of oxidative free radical scavenging enzymes and lipid peroxidation in rat frontal cortex and striatum. J Ethnopharmacol 74, 1-6.
About Author / Additional Info:
I am scientist at Crop Protection Division, ICAR-Indian Institute of Wheat and Barley Research, Karnal, Haryana